1. Introduction
2. Wind-Turbine
3. Support Structure
3.1: Gravity Base Structures [GBS]
3.2: Monopile
3.3: Tripod
3.4: Floating
3.5: Design Calculations
4. Windfarms
4.1: Grid Integration
4.2: Economics
5. Summary and Conclusions
6. References
7. Acknowledgements
Figure 1: Middelgrunden Windfarm
Offshore wind farms promise to become an important source of energy in the near future: it is expected that by the end of this decade, wind parks with a total capacity of thousands of megawatts will be installed in European seas. This will be equivalent to several large traditional coal-fired power stations. Plans are currently advancing for such large-scale wind parks in Swedish, Danish, German, Dutch, Belgian, British and Irish waters and the first such parks are currently being constructed at Horns Rev, off Denmark's western coast and Rødsand, in the Danish part of the Baltic coast.
Onshore wind energy has grown enormously over the last decade to the point where it generates more than 10% of all electricity in certain regions (such as Denmark, Schleswig-Holstein in Germany and Gotland in Sweden). However, this expansion has not been without problems and the resistance to windfarm developments experienced in Britain since the mid 1990s, is now present in other countries to a lesser or greater extent. One solution, of avoiding land-use disputes and to reduce the noise and visual impacts, is to move the developments offshore, which also has a number of other advantages:
* availability of large continuous areas, suitable for major projects,
* higher wind speeds, which generally increase with distance from the shore (Britain is an exception to this as the speed-up factor over hills means that the best wind resources are where the turbines are also most visible),
* less turbulence, which allows the turbines to harvest the energy more effectively and reduces the fatigue loads on the turbine,
* lower wind-shear (i.e. the boundary layer of slower moving wind close to the surface is thinner), thus allowing the use of shorter towers.
But against this is the very important disadvantage of the additional capital investment necessary, relating to:
* the more expensive marine foundations,
* the more expensive integration in to the electrical network and in some cases a necessary increase in the capacity of weak coastal grids,
* the more expensive installation procedures and restricted access during construction due to weather conditions,
* limited access for O & M during operation which results in an additional penalty of reduced turbine availability and hence reduced output.
However the cost of wind turbines is falling and is expected to continue doing so over the coming decade and once sufficient experience has been gained in building offshore projects, the offshore construction industry is likely to find similar cost-savings. At locations with good windspeeds, onshore wind energy has become a cost-competitive resource at a stable price compared to conventional power generation, especially when environmental benefits are accounted for. Hence it would seem likely that offshore wind energy will also become competitive in time. Other developments that are likely to support this trend are the design of turbines optimised for the offshore environment, of greater sizes (maybe up to 10 MW and over 125 m rotor diameter eventually) and with greater reliability built-in. At the moment, the largest production machines have generating capacities of 2.5 MW but prototypes of machines with power outputs of up to 5 MW are currently being constructed, and with full-scale serial manufacturin
g generally a couple of years behind, the middle-of-this decade should allow a developer to choose between several competing machines. The wind turbine manufacturing industry has been following its own exponential growth curves over the last decade of decreasing costs by 20%, increasing annual installed capacity by 50% and doubling the size of the largest commercially-available turbine every three or so years.
The total wind power resources available offshore are vast and will certainly be able to supply a significant proportion of our electricity needs in an economic manner. Several previous studies, including those by Garrad Hassan / Germanischer Lloyd [11] and EWEA / Forum for the Future [10] have concluded that a large proportion of Europe's power could be supplied from offshore wind turbines.
Table I shows how the installed capacity of offshore windenergy might develop over the next few years. These figures are based on announcements by developers and government ministers and hence are subject to amendment; the chances of these figures turning out to be correct must be very low - whether they are an under or an over estimate is not clear.
Table I: Prospective Expansion of Offshore Windfarm Capacity
Country
|
2001
|
2002
|
2003
|
2004
|
Declared
|
Target / Estimated
|
Denmark
|
50
|
230
|
400
|
600
|
900
|
900
|
Sweden
|
23
|
23
|
23
|
137
|
137
|
137
|
Netherlands
|
19
|
19
|
130
|
260
|
260
|
6,000
|
Ireland
|
-
|
-
|
60
|
120
|
770
|
770
|
Germany
|
-
|
-
|
60
|
60
|
5,600
|
5,600
|
UK
|
4
|
4
|
210
|
800
|
1,800
|
1,800
|
France
|
|
|
|
|
1,500
|
1,500
|
Total
|
96
|
276
|
883
|
1,977
|
10,967
|
16,707
|
|
A Brief History
|
|
One of the first wind turbines to generate electricity was a traditional wooden windmill converted by Poul la Cour in Denmark over 100 years ago. In the early part of the 20th century, there were further experimental machines but serious developments only began with the two oil shocks in the 1970s, when governments around the world reacted by directing R&D money to alternative fuel sources. The early '80s saw major developments in California and the construction of the famous fields of hundreds of small turbines and by the end of that decade there were 15,000 turbines with a total generating capacity of 1,500 MW in that state [1]. The stabilising of the oil price in the '80s and resulting reductions in the state subsidies for windpower, meant that purchases from the crucial American market dried up and many wind turbine companies withdrew from the field or went bankrupt. An exception was in Denmark, where government support meant that the knowledge base was not dissolved and the companies there were able |
to quickly respond when wind energy's fortunes revived once more in the early '90s, to the point where they and their partners continue to have a strong position in the market today. It should be pointed out that the foundations of renewable energy's fortunes are today based on the solid necessity of alleviating climate change and increased energy autonomy rather than the fickle nature of oil prices.
|
|
|
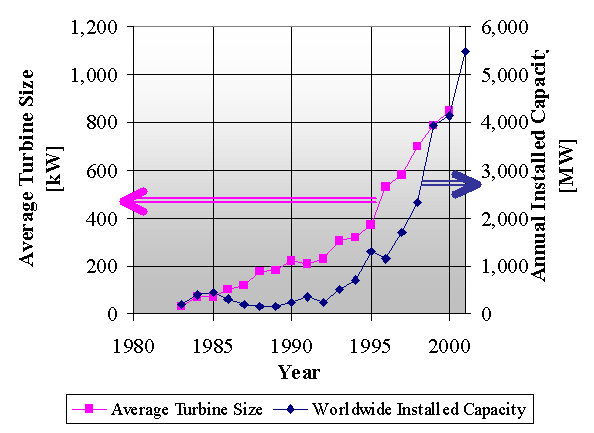
leeg |
Figure 2: Size & Installation Trends [4]
|
|
Currently there is a total installed capacity of approximately 24 GW on land and last year the annual installation rate reached 5.5GW/annum. The average rating of turbines being installed is now approaching 1MW per unit internationally, Figure 2. With the resulting economies of scale, wind energy now competes on price with the traditional generators, such as coal and nuclear, in areas of rich wind resources.
|
|
leeg |
2. Wind-Turbine
leeg |
leeg |
The wind turbines being used in current offshore projects tend to be machines designed for land-use but with modifications, such as a larger generator, a higher instrumentation specification and component redundancy, particularly of electrical systems. If the market expands as expected, machines designed for optimised performance offshore will be developed and utilised but it is not certain how they will look. On one hand, the requirements from an offshore machine differ from those on land, however the requirement for high reliability would suggest the use of well-proven turbines. Modifications may include:
|
* larger machines, up to 5 MW or 10 MW,
|
* faster rotational speeds than on land, where noise restrictions generally mean that the turbine operates slightly below optimum speed,
|
* larger generators for a specific rotor size, to enable the additionally available energy to be efficiently harvested,
|
* high voltage generation, also possible in DC instead of AC,
|
|
In the longer term, downwind machines with flexible blades or multiple rotors might become an option, but engineering effort will be needed to achieve the claimed theoretical potential.
|
|
Table II illustrates the increase in rotor speed that has been designed into machines developed specifically for the offshore market. It can be seen that tip speed of the offshore machine has been increased in all cases shown here, by between 10% and 33%, the associated small increases in noise levels being unimportant at offshore locations.
|
|
Table II: Trends in tip speed comparing offshore and land based turbines
|
Wind Turbine
|
Rated Power [kW]
|
Control concept
|
Tip speed [m/s]
|
Ratio of Tip-Speeds (offshore/land)
|
Vestas V66 (land)
|
1650
|
Pitch reg., variable slip
|
66
|
-
|
Vestas V80 (offshore)
|
2000
|
Pitch reg., variable speed
|
80
|
1.21
|
Nordex N60
|
1300
|
Stall reg., fixed speed
|
60
|
-
|
Nordex N80 (offshore)
|
2000
|
Pitch reg., variable speed
|
80
|
1.33
|
Bonus 1300 (land)
|
1300
|
Active stall, fixed speed
|
62
|
-
|
Bonus 2000 (offshore)
|
2000
|
Active stall, fixed speed
|
68
|
1.10
|
NEG Micon 1000/60 (land)
|
1000
|
Stall reg., fixed speed
|
57
|
-
|
NEG Micon 2000/72 (offshore)
|
2000
|
Active stall, fixed speed
|
68
|
1.19
|
The economics of offshore wind energy encourage the development of very large wind-turbines in order to justify the additional investment necessary for the more expensive support structures, grid connection and installation procedures. Over the next couple in years, a handful of prototypes are going to be constructed, ranging in size up to over 110m diameter and 5 megawatts rated power, see Table III. The economics will become particularly important in the deeper waters of interest to German developers and in the longer term elsewhere as well when the most suitable near-shore sites have already been developed or visual-impact becomes an important issue.
Table III: Future MultiMegaWatt Machines
Manuf.
|
Power
|
Diam
|
Location
|
Prototype
|
GE Wind
|
3.6 MW
|
100m
|
Spain
|
Apr 02
|
Enercon
|
4.5MW
|
112m
|
Magdeburg, DE
|
mid '02
|
Vestas
|
3 MW
|
90m
|
Friesland, DE
|
May '02
|
NEG Micon
|
2.75 MW
|
90 m
|
Orkney, UK & ECN, NL
|
Mid & Late '02
|
Nordex
|
5 MW
|
E115m
|
-
|
'03
|
REPower
|
5 MW
|
E115m
|
-
|
'03
|
Pfleiderer
|
5 MW
|
E115m
|
-
|
'04
|
DeWind
|
3.5 MW
|
E90m
|
-
|
'03
|
Costs: Under conditions of true similarity in design style, state of technological progress and design specification, costs of large turbines might be expected to scale cubically with rotor diameter. Considering however historical data over the range of machine sizes, ongoing technology development results in a scaling closer to a square law than a cubic law. Price data of onshore machines show a gently rising unit cost (per m2 swept area) for rotor diameters of 40 m and greater, Figure 3, although this could partly reflect the fact that smaller turbines were generally introduced to the market longer ago and with the development costs previously having been recouped, the selling-price can be reduced. Although marinisation of onshore design generally adds 10% in costs, the currently available offshore specific machines are now essentially on lower cost curves than onshore predecessors.
Figure 3: Relationship between Turbine Unit Costs [per m2] and Rated Power
Blade technology: The demand for high strength blades of low solidity in conjunction with diminishing carbon fibre costs is driving the industry in the direction of carbon epoxy. Carbon prices are falling and if it were used in significant quantities in blades for offshore machines, that could become by far the largest market for high quality carbon fibres, thus resulting in further cost reduction.
Gearbox: It is not clear whether the current gearbox concept (three stage units, input stage planetary, the two higher speed stages parallel with helical gears) will be applicable for larger, offshore turbines, since it is likely that for larger machines, i.e. > 3MW, an additional gearbox stage will be required, resulting in increased complexity and probability of failure. This may be an important driver towards direct drive systems.
Variable speed: There is a tendency towards variable-speed designs. Wide range variable speed has a further advantage in the ability to avoid damaging resonances, important for offshore turbine structures, where the resonant frequencies have proved difficult to predict accurately, and may also change over the lifetime of the structure. It remains somewhat unclear whether power electronic converters can be made reliable enough at suitable cost.
So far, most offshore wind farms have been built using gravity base structures, when in very shallow waters (i.e. below about five metres depth) and monopiles, when in medium depth waters, (i e greater than 5 water depth).
3.1
Gravity Base Structures [GBS]
Gravity base structures (GBS) have been used at the first three Danish offshore windfarms with Figure 4 showing the type as used at Middelgrunden. The structure has a large flat base, to resist overturning forces imposed by the turbine rotor, the size of which will depend on the wave climate and the ground conditions. At the water surface level, an ice-breaking cone is shown, as would be necessary in the less salty waters of the Baltic Sea. The water surface level must be at the level of the inclined surface of the cone at all tides, including storm surges, which also matches Baltic Sea conditions. Ice cones bring a benefit of reduced ice-loading by causing the ice sheets to bend downwards and break-up as they make contact with the wind-turbine however the greater structural size at the water surface also means that wave loads are increased and more difficult to predict.
Figure 4 Gravity Base Support Structure
The current design philosophy for wind farms in water depths up to 20 m is based on the monopile with the installation methodology (driving, drilling or combination) depending on soil properties, water depth and contractors experience. Monopiles are a relatively compliant structure and hence are more difficult to design; uncertainties in ground conditions for example can result in a structure with a quite different structural frequency than designed for, with all the potential resulting problems of resonance-induced dynamic oscillations. For this reason, the cost benefits of designing a less rigid support structure may not be considered worthwhile by the developer.
Figure 5: Monopile Support Structure
For deeper waters, tripod support structures are being considered but the optimum solution is not yet certain and may well be a concept currently being brought into the field by offshore and coastal engineering specialists. There has already actually been one offshore wind-turbine constructed on a tripod, the first wind-turbine built at Nogersund in Sweden; however this was a small turbine located in shallow water and hence unlike the structures to be built in the future.
Figure 6: Tripod Support Structure
Figure 7: Alternative Tripod Support Structure
Potential for floating wind energy is going to depend whether the current cost disadvantages can be overcome by the development of innovative solutions to constructions and installation. Countries in North Europe are fortunate in that many have significant stretches of shallow water near to their shore and close to the demand centres and these will be the first to be developed. The USA and Canada also have suitable shallow regions however other regions, such as Japan, do not. [12]
Figure 8: Candidate Floating Support Structure - Based on Spar Buoy Concept
Designing a flexible structure can result in benefits of reduced loads and hence reduced costs, however this generally results in fatigue become the design driver, which is more difficult to analyse. The trend towards more flexible structures can be characterised by the relationship between the frequency of the tower's first bending mode and the frequency of the main loads: the rotor, the blade-passing (of the tower) and the waves. For some of the more recently constructed offshore and onshore windfarms, the tower has been designed so that its natural frequency reduces to close to and has even be in between that of the blade and rotor loads (the 1P and 3P loads for three-bladed rotors).
Figure 9: Compliance of the Structure
Figure 10: Large Future Offshore Windfarm
Operation and Maintenance (O & M) of offshore wind farms is more difficult and expensive than for equivalent onshore wind farms. The current reliability and failure modes of commercial offshore wind turbines are such that a "no maintenance" strategy is not a viable option. Improved preventive and corrective maintenance schemes will become crucial for economic exploitation of offshore wind power. In particular improving the accessibility is a key factor in increasing availability [5]. A number of current projects are attempting to address the issue of improved access to offshore wind turbine installations: with most focusing on maintaining existing boat access methods with emphasis on addressing the issue of motion compensation or complete removal of the vessel from the water at the turbine location. Suggested improvements to the base of OWECS to facilitate safe personnel access include: fixed platforms, flexible gangways, friction posts against which the vessel maintains a forward thrust during t
ransfer, vessel lifting facilities and winch / netting for personnel and equipment (eliminating the need for specialist lifting vessels for major component replacement).
The issue of availability should also be addressed through improvements in offshore wind turbine reliability. Unplanned maintenance levels can be reduced by increasing the reliability of the turbine. Particular emphasis is being placed on reliability issues from overall design improvements through to component level. Designing for reduced maintenance could drive wind turbine designs away from current onshore standards, such as towards two bladed configurations, direct drive technology or application of electrical actuators; however use of unproven technologies is intrinsically less reliable than proven systems.
Figure 11: Importance of Reliability for Offshore Wind Turbines
Electrical systems: There are many areas where technical developments in electrical systems are expected which will improve the economics and reliability of offshore wind farms. Some of these will arrive because of developments in other industries and in onshore wind, but others are specific to offshore wind and are therefore more risky. Developments can be expected to take place within the wind turbine (such as generation at high voltage) and with in the wind farm electrical systems, regarding set-up of substations, use of HVDC technology and cable technology.
In the past, grid operators have had to deal with stochastic variation of the power demand only but wind and some other renewables introduce stochastic variation into the supply as well. Up to a fairly high penetration level into the national supply (in the order of 10%), the power can generally be absorbed without too much difficulty, as in principal, stochastic mismatch is the same problem, whichever side it originates from. Beyond that, non-dispatchable generators pay a price penalty, if additional conventional reserve power is required to ensure the balance between production and consumption. This can be reduced by using wind-forecasting to predict the output further in advance or by matching with dispatchable but limited sources of power, such as hydro-electric. The coming decade is likely to see the expansion of several previously non-conventional electricity generation technologies, including micro-generation [9], hence grid operators will have an incentive and pressure from several generator-tech
nologies to react positively to these challenges. In addition, integrating large offshore windfarms into the electricity grid may pose problems if the coastal network is weak and more advanced power control systems may be used to conform with grid connection requirements. This may provide opportunities for the application of new technologies for electricity storage combined with more reliable models for predicting fluctuations from wind energy over longer periods.
Cross-border power transmission limitations prevent a geographical smoothing of the production/consumption imbalance. Solutions to deal with large imbalances are: Demand Side Management, increased flexibility and dispatching capability of conventional plants, the use of energy storage, application of wind power forecasting techniques and increasing the controllability of wind farm output. It is concluded that, although all options could eventually contribute to the solution (requiring much more RTD), the most promising immediate step is to increase the accuracy and reliability of wind power forecasting techniques.
The impact of large-scale offshore wind power on power systems performance (power quality) requires special attention since coastal connection points will often be relatively weak. Flicker, harmonics and interharmonics and static stability are not considered as limiting factors but dynamic grid stability may be a limiting factor, in particular in relation to wind farm correlated sudden shut-down of wind turbines. These problems may lead to modifications in wind turbine control philosophies for the high wind speed cut-out.
Large scale offshore wind power will further impose an increase of primary control (response time of the order of seconds) and secondary control (response time of the order of minutes) requirements of the conventional production components of the system; such requirements could also be imposed on large wind farms, although it remains unclear how such requirements could be efficiently implemented.
The connection technology between offshore wind farms and the grid is characterised by large power (> 100 MW) and potential long distances. HVDC links offer a possible lower-cost solution, which could also contribute to alleviate the power quality management problems mentioned above.
Access of large offshore wind farms to the grid must be in accordance with national grid codes. Current requirements imposed by national grid codes are in general not considered to be a limiting factor for the development of large-scale offshore wind power, although these requirements are not particularly suitable for non-predictable, highly variable energy sources. Project developers may have to take additional measures to comply with the grid codes, such as: use of variable speed wind turbines, special purpose remote control systems (with individual power set points for the wind turbines, etc). In the long term, HVDC transmission and/or on-site large storage facilities with controllable reactive power output, might present interesting opportunities allowing large scale offshore wind power plants to meet grid access requirements more easily to the point of possibly having a positive impact on grid stability.
Offshore projects require initially higher investments than onshore due to turbine support structures and grid connection. The cost of grid connection to the shore is typically around 25% a much higher fraction than for connection of onshore projects. Other sources of additional cost include foundations (up to 30%), operation and maintenance (with expected lower availability) and marinisation of turbines, Figure 12 shows a possible distribution of the capital investment costs however the water depth and distance to shore can have a significant impact on redistributing the costs. Note that the O & M costs are excluded and these will probably typically amount to around a quarter of the Levelised Production Costs (LPC). The similar magnitudes of cost for several different components (wind-turbine, support structure, power collection & transmission and O & M) emphasises the importance of an integrated approach to the design of the whole windfarm development.
Investment costs have been reduced from about 2200 /kW for the first Danish offshore wind farms to an estimated cost of 1650 /kW for Horns Rev (equivalent to 4.9 c/kWh). This compares with typical figures for onshore sites of investment 700-1000 /kW and estimated energy cost of 3-8 c/kWh for a mean wind speed of 5-10 m/s.
Figure 12: Typical Distribution of Costs for Offshore Windfarm
Financing: From the current developments of demonstration offshore projects of various sizes, it would appear that sufficient equity capital is available for financing offshore wind farm projects. Some major oil & gas companies and utilities have announced projects, which could be financed by company equity. However it still remains to be determined under which conditions (due diligence, certification, insurance etc) bank loans will be granted for offshore wind farm projects. Only test and demonstration projects will provide information to allow an answer to this question. At least they will reduce the present uncertainties related to the cost of energy generated. Important support comes from a variety of national incentive mechanisms, such as investment subsidies, tax exemptions, fixed tariffs and green certificate schemes.
The distribution of the investment costs for an onshore windfarm show a much heavier focus on the windturbine as illustrated by a typical example in Figure 13.
Figure 13: Typical Distribution of Costs for Onshore Windfarm
It would seem that the current optimism about offshore windenergy has a firm basis, in currently available technology, in likely reductions in cost and of equal importance, in the general widespread public and political support and the generally low impact on the environment. The experience of the first prototype offshore windfarms has proven the technical viability and the large-scale developments currently being undertaken will bring in much practical experience on issues such as construction methods, installation procedures, access and O & M philosophies which will result in a more economic generation cost of electricity.
[1] Ackermann, T. & Söder L., Wind energy technology and current status: a review, Renewable & Sustainable Energy Reviews, Vol. 4, 2000.
[2] Barthelmie, R. A Brief Review of Offshore Wind Energy Activity in the 1990's, Wind Engineering, Vol. 22, No. 6, p 265, 1998.
[3] Border Wind for Greenpeace. "Offshore Wind Energy. Building a New Industry for Britain." June 1998.
[4] British Wind Energy Association, 26 Spring Street, London W2 1JA, Tel 020 7402 7102, http://www.britishwindenergy.co.uk/, 2001
[5] van Bussel, G.J.W., and Zaaijer, M., Reliability, availability and maintenance aspects of large-scale offshore wind farms, a concept study, Marine Renewable Energies Conference [ MAREC ], Newcastle, UK, 2001
[6] Clausager, I.: Impact assessment studies of offshore wind parks on seabirds with special reference to the TunEKnob Park, in: Merck & von Nordheim: Technische Eingriffe in Marine Lebensräume, Tagungsband. BFN-Skripten 29. Bundesamt fE Naturschutz, 2000.
[7] Danish Wind Turbine Manufacturers Association (DWTMA); Wind Windpower No. 2 EMarch 1995 1 Note; No. 2 March 1996; Employment In the Wind Power Industry, 1996
[8] DLR/WI/ZSW/IWR/Forum: "Klimaschutz durch Nutzung erneuerbarer Energien", Final Report, 2000
[9] The Economist, The dawn of micropower, Aug 5th 2000.
[10] Forum for Energy and Development, "Windforce 10" A blueprint to achieve 10% of the world's electricity from wind power by 2020, www.inforse.org, 2000
[11] Germanischer Lloyd and Garrad Hassan, Study of offshore wind energy in the EC, ETSU Report W/35/00250 and Publ. Verlag NatEliche Energie JOUR 0072, both 1994.
[12] Henderson, A. R., Leutz, R., Fujii, T., Potential for Floating Offshore Wind Energy in Japanese Waters, ISOPE Conference, 2002
[13] KEn M, Ferguson M.C., Göransson B., Cockerill T.T., Harrison R., Harland L.A., Vugts J.H., Wiecherink R., 'Opti-OWECS; Structural an Economic Optimisation of Bottom Mounted Offshore Wind Energy Convertors', JOULE III project, Delft University of Technology, Vols. 0-5, Delft, Netherlands, August 1998.
[14] Sørensen, H. C. et al, Middelgrunden 40 MW offshore wind farm, a prestudy for the Danish offshore 750 MW wind program, ISOPE Conference Seattle, 2000.
[15] SEAS, Havvindmøller VVM. Støjundersøgelse - undervandsstøj. [Offshore wind turbines EIA. Noise investigation - underwater noise], 2000
[16] SEAS, Rødsand Offshore Wind Farm. Environmental Impact Assessment. EIA Summary Report. Summary reports in English and German, full report in Danish and background reports available from www.seas.dk, 2000
[17] Sundberg, J. & Söderman, M, "Windpower and grey seals: An impact assessment of potential effects by sea-based windpower plants on local seal population." Department of Animal Ecology, Uppsala University, 1999
Acknowledgements are due to the contributions from the partners within the Concerted Action on Offshore Wind Energy in Europe [CA-OWEE] project on which parts of this article are based.
|
Webcontent © 2002 www.offshorewindenergy.org
|
Updated September 2008
|